Reaching a New Level in Dark Matter Searches
Jan Conrad, Stockholm University
Over the last couple of years, the evidence for the existence of a significant component of matter in the universe that cannot be viewed with conventional telescopes has become indisputable – this component is known as Dark Matter (DM). Present measurements indicate that only a few percent of the total matter in the universe is made of particles that we know about. The nature of DM however is completely unknown, though many theoretically motivated explanations have been proposed. The most popular theory is that DM consists of a new type of elementary particle created in the early Universe, which has been dubbed the weakly interacting massive particle (WIMP). These particles would interact very feebly but would be a hundred to a thousand times heavier than a proton. Swedish scientists from Stockholm University are now participating in one of the leading experiments worldwide to search for these particles. This experiment is called XENON1T and is based at the Laboratori Nazionali del Gran Sasso (LNGS) in Italy. LNGS is one of the largest underground laboratories in the world. It is situated under about 1.4 km of rock which provides necessary protection against background interference induced by cosmic ray interactions as well as a unique infrastructure for the challenging search for WIMPs. The XENON1T dark matter xenon detector was built and is operated by a collaboration consisting of research groups from 21 institutes in France, Germany, Italy, Israel, the Netherlands, Portugal, Sweden, Switzerland, the United Arab Emirates and the USA. In total about 130 physicists are involved in the project.
Apart from leading a variety of tasks within the experiment, Sweden hosts the European analysis hub, a computer cluster provided by PDC, which is partly financed by the Knut and Alice Wallenberg Foundation.
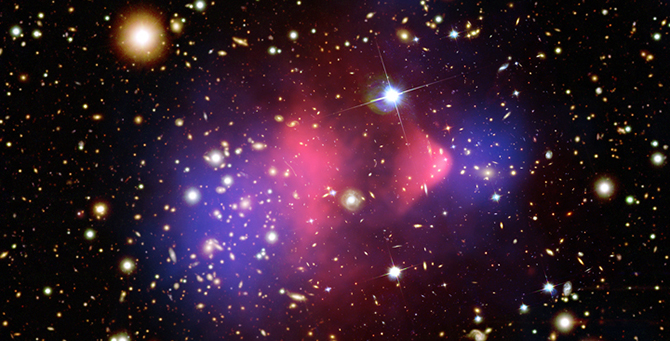
The idea of the dark matter detector is to attempt to detect the recoil of nuclei that WIMPs interact with. The challenge is that, even in one tonne of heavy liquefied noble gas, we only expect one or two of these WIMPs to recoil per year. This would be alright, if it was not for the more than 100 billion other interactions that we need to filter out. These pose a formidable challenge in terms of computing, data handling and data reduction. The amount of data that is collected from the detector can, at times, be as much as 300 MB per second, and in total we expect to collect approximately 6 PB of data during the next few years.
The dark matter detector is made of xenon gas that is kept at -95 °C, which means that the gas is liquefied. Any nucleus that recoils (due to colliding with a dark matter particle) causes ionization and excitation of the surrounding xenon atoms. The resulting freed electrons are drifted in an electric field to the top of the detector where xenon is kept in gas phase – as there they will cause an avalanche of electrons. Eventually in this way two signals should be produced: one from the interaction with the gas phase xenon and one from interacting with the liquid phase xenon. Both signals would be detected by arrays of photo-multiplier tubes (PMTs) which are light sensors that are sensitive to single photons. This concept is known as a time projection chamber (TPC). The fact that each such scattering produces two signals can then be used to separate the precious WIMP-induced recoils from all the noise that is caused by the much more abundant background consisting of cosmic rays and the radioactivity that is in the environment and in the detector components themselves. See the figure for an illustration of the concept.
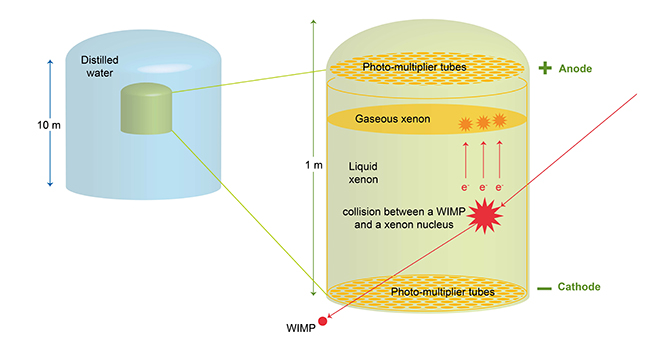
The XENON1T detector is about 30 times larger than its predecessor, consisting of about 3.5 tonnes of liquid xenon in total. The XENON1T TPC, which is a cylindrical shape 1 m high and 1 m in diameter that is laterally encased by highly reflective Teflon, is the largest liquid xenon TPC ever operated. The XENON1T TPC will host 2 tonnes of liquid xenon (LXe) in two arrays consisting of a total of 248 3’’ PMTs, with 121 PMTs at the bottom immersed in LXe, and 127 PMTs on the top in the gaseous phase xenon. The figure shows a picture of the TPC.
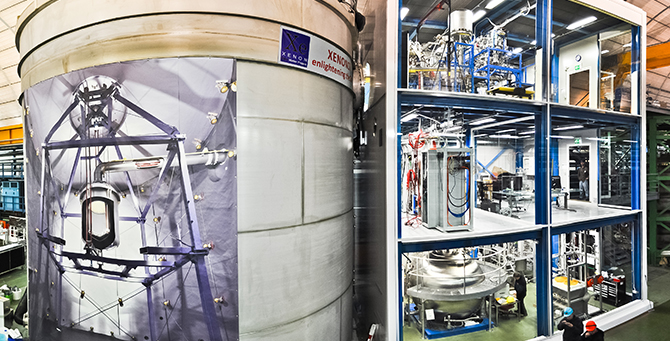
As we mentioned earlier, being able to separate out and reject the data relating to background events is the key to success for direct detection experiments. The backgrounds induced by the environment and cosmic rays can be minimized by exploiting the self-shielding capabilities of the xenon. Most external particles stop after a few centimetres in the heavy xenon material. In addition, the XENON1T detector is surrounded by a cylindrical water tank, 10 m high and 9.6 m in diameter, that is equipped with PMTs as an additional shield against cosmic rays. For a detector the size of XENON1T, radioactive impurities in the detector parts and the xenon itself become the biggest challenge for reducing background noise. Extensive radiation screening campaigns, using some of the world’s most sensitive germanium detectors, were conducted and high purity PMTs have been specially developed by the XENON1T collaboration. Contamination of the xenon by radioactive radon (mainly 222Rn) and krypton (85Kr) lead to the development of cryogenic distillation techniques, which mean that the abundance of these isotopes is suppressed to levels below a few parts per trillion (in the case of krypton).
The XENON1T detector was inaugurated on the 11th of November 2015 and we are about to finish work on commissioning and calibrating the detector. At the time of writing, we are about to start the first data collecting period looking for the rare dark matter interactions. After only about two months of continuous running, we will have probed the existence of WIMP dark matter with hitherto untested properties, which is predicted by the most favoured theories. A detection is clearly in the cards, especially considering a relatively simple upgrade that we are already preparing, and that we expect will start operations by 2019. This upgrade will enlarge the detector to almost 8 tonnes of xenon.
In addition to the experimental work, the interpretation of the results requires advanced techniques from theoretical particle physics and nuclear physics. To provide this necessary progress to the international community, a consortium of researchers from Stockholm University and theorists from Chalmers Technical University has been formed. The consortium has been dubbed SweDCube and is supported the Knut and Alice Wallenberg Foundation. It is certain that this interpretation work will also require advanced numerical calculations where PDC’s computing resources will be helpful.
Within the next five to ten years we should be in a position to detect dark matter if the current paradigm is correct. If not, we will face a paradigm shift and will have to rethink our approach to dark matter. With the help of PDC, Swedish scientists will be in the front line of this endeavour.